Ultrafast switching with light in correlated materials
Photo: Peter Kraus, credits: Ivar Pel
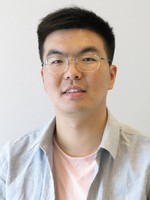
In strongly correlated materials, interaction of the electrons within the material leads to unexpected properties. Postdoc and first author Zhonghui Nie explains: “We study a class of strongly correlated materials that are compounds of oxides. Theory predicts these compounds to be conducting, like a metal, but they actually behave like an insulator most of the time. However, above a threshold temperature they undergo a phase transition and switch to being conductive.”
Light-based switch for micro-electronics
The properties of these materials are still not fully understood, but their switching behavior makes them interesting for the semiconductor industry. Because oxide electronics could potentially reduce the energy consumption of the industry, this class of correlated materials is investigated by many groups in the field.
“These materials switch to a conductive phase when their electrons get more freedom to move,” says Nie. “This happens above a threshold temperature, but can also be induced by a light pulse, when photons excite electrons which weakens electron interaction inside the material. Therefore we can potentially use light as a switch. Meanwhile, light pulses can also heat the sample. This means that for correlated materials with a low threshold temperature – not much above room temperature – it is hard to discern whether a light pulse causes it to switch due to a change in electron interaction or due to heating of the sample.”
New approach to high-harmonic generation
To overcome this problem, Nie and his colleagues are studying a polycrystalline thin film of the compound niobium dioxide, which has a high threshold temperature of over 700 K. They use a new method to study nanostructures, for which group leader Peter Kraus received an NWO Vidi grant in June 2023.
The current standard to study very small structures with light is to use a very short wavelength, i.e. smaller than the relevant dimensions in the sample (~ 100 nm).
Such ultra-short wavelengths are obtained in a process called ‘high-harmonic generation’, a topic for which the Nobel Prize was awarded recently. In this process a light pulse with a longer wavelength is directed at a gas, which results in interference and non-linear optical effects causing the light to split into several higher order waves, with a much shorter wavelength: the high harmonics. These high harmonics can then be used for spectroscopy of nanostructures.
Nie’s and Kraus’ approach is to generate these high harmonics directly from the solid sample under study, instead of in a separate gas. Nie used this new method to study the switching behavior of correlated materials.
“We wanted to know if we could trigger the phase transition with light, and also show that there are no thermal effects,” says Nie. “With the high-harmonic generation as a probe method, we found that our material switched from the insulating to metallic phases at an unprecedented fast time scale of 100 fs. The light pulse is short and has too little energy to induce enough heating in the sample for a phase transition. So we know that the transition is caused by light manipulating the correlated electrons, and not by heating.”
Neural networks
The fast response time in Nie’s experiments holds great promise for opto-electronic manipulation of materials at the ultrafast time scales required in microelectronics. “Using correlated materials in a future computer would require the switching to be even a bit faster, but if that could be achieved, oxide electronics can potentially make the semiconductor industry a lot greener because it will need much less energy than current technology,” says Nie. “This type of switching is also promising for neuromorphic engineering and artificial intelligence, because electronic elements based on phase transition can realize nonlinear electronic responses and mimic neural networks.”
Reference
Z. Nie et al., Following the Nonthermal Phase Transition in Niobium Dioxide by Time-Resolved Harmonic Spectroscopy, Phys. Rev. Lett. 131, 243201 (2023).
https://doi.org/10.1103/PhysRevLett.131.243201